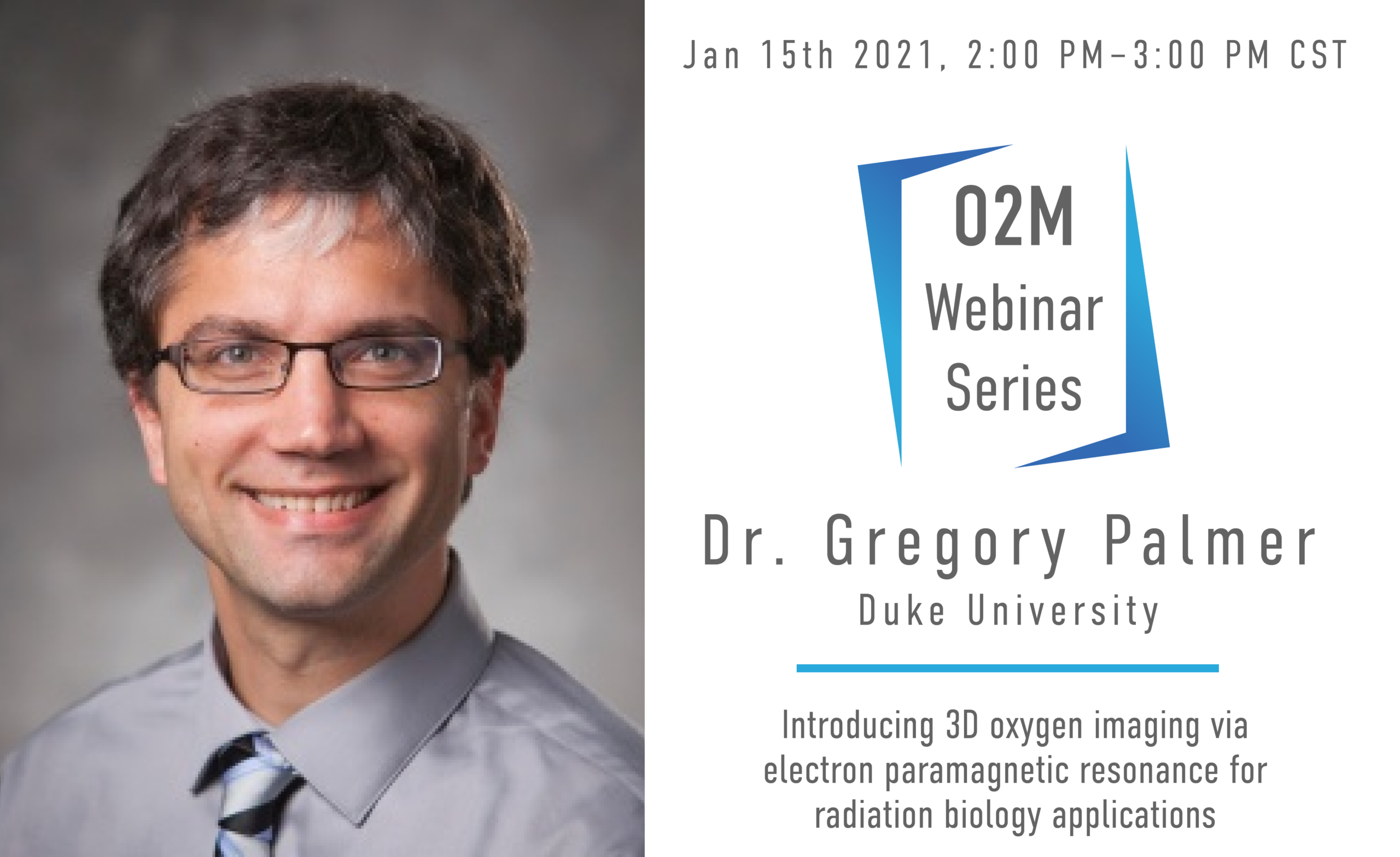
Moderated By: Dr. Mark Dewhirst, Duke University
About the Speaker: Greg Palmer obtained his B.S. in Biomedical Engineering from Marquette University in 2000, after which he obtained his Ph.D. in BME from the University of Wisconsin, Madison. He is currently an Associate Professor in the Department of Radiation Oncology, Cancer Biology Division at Duke University Medical Center. His primary research focus has been identifying and exploiting the changes in absorption, scattering, and fluorescence properties of tissue associated with cancer progression and therapeutic response. To this end he has implemented a model-based approach for extracting absorber and scatterer properties from diffuse reflectance and fluorescence measurements. More recently he has developed quantitative imaging methodologies for intravital microscopy to characterize tumor functional and molecular response to radiation and chemotherapy. His awards have included the Jack Fowler Award from the Radiation Research Society.
About the Webinar: Hypoxia severely limits the efficacy of radiotherapy, chemotherapy, and immunotherapy. This results in diminished success in treating cancer. Despite consistent research in this field and treatments with promising anti-hypoxia mechanisms, there is no treatment for ameliorating hypoxia. This could be a result of ineffective hypoxia mitigators or because there is not a clinical imaging modality capable of directly quantifying oxygen at high temporal, spatial and oxygen resolution. Electron paramagnetic resonance oxygen imaging (EPROI) offers 3D oxygen maps at high resolution. The first, commercial, preclinical EPROI unit, JIVA-25 developed by O2M, is undergoing its final validation. At Duke University, this EPROI system is currently being utilized in radiation biology applications, investigating a promising radiation sensitizer in the E0771 murine flank tumor model. Oxygen microbubbles are venously-introduced and burst in the tumor via ultrasound, providing a bolus of oxygen. Preliminary in vitro data reports that an increase in tumor pO2 mere milliseconds before radiation significantly increases radiation-induced cancer cell damage. In vivo pilot studies have shown an increase in hemoglobin saturation in murine tumor models; however, to fully elucidate the role of oxygen microbubbles in alleviating hypoxia and to determine the ideal timing of prospective radiotherapy, the increase in tissue pO2 must be directly quantified spatially and temporally. We hypothesize that oxygen microbubbles will cause an acute increase in tumor pO2. Here, we present our initial data from this study. These described experiments, while focusing on the radiation biology field, will provide a preclinical imaging paradigm for quantifying hypoxia in vivo that is applicable to any research that requires precise evidence of tissue pO2.